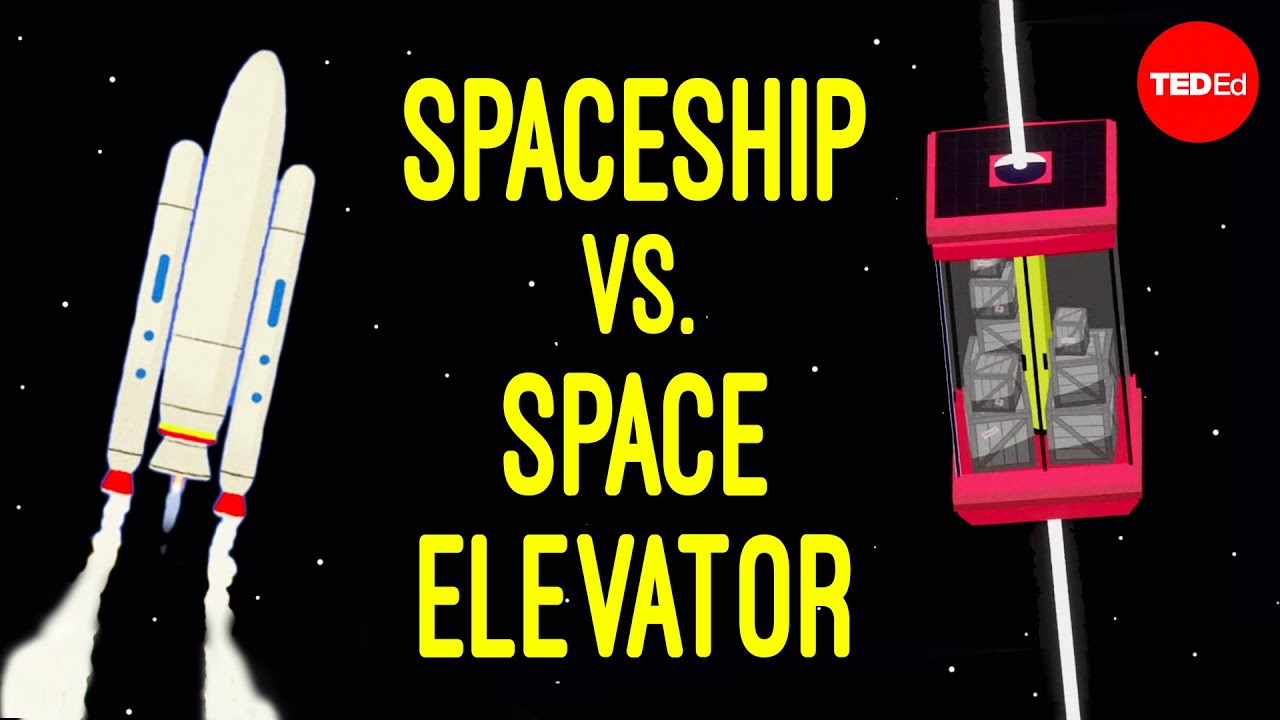
Yes, scientists are actually building an elevator to space - Fabio Pacucci
Sending rockets into space requires
sacrificing expensive equipment,
burning massive amounts of fuel,
and risking potential catastrophe.
So in the space race of the 21st century,
some engineers are abandoning rockets
for something much more exciting:
elevators.
Okay, so maybe riding an elevator
to the stars
isn't the most thrilling mode
of transportation.
But using a fixed structure to send
smaller payloads
of astronauts and equipment into orbit
would be safer, easier, and cheaper
than conventional rockets.
On a SpaceX Falcon 9 rocket,
every kilogram of cargo costs
roughly $7,500 to carry into orbit.
Space elevators are projected
to reduce that cost by 95%.
Researchers have been investigating
this idea since 1895,
when a visit to what was then
the world's tallest structure
inspired Russian scientist
Konstantin Tsiolkovsky.
Tsiolkovsky imagined a structure
thousands of kilometers tall,
but even a century later,
no known material is strong enough
to support such a building.
Fortunately, the laws of physics offer
a promising alternative design.
Imagine hopping
on a fast-spinning carousel
while holding a rope attached to a rock.
As long as the carousel keeps spinning,
the rock and rope will remain horizontal,
kept aloft by centrifugal force.
If you're holding the rope, you'll feel
this apparent, inertial acceleration
pulling the rock away from the center
of the rotating carousel.
Now, if we replace the carousel
with Earth,
the rope with a long tether,
and the rock with a counterweight,
we have just envisioned
the modern space elevator—
a cable pulled into space
by the physics of our spinning planet.
For this to work, the counterweight would
need to be far enough away
that the centrifugal force
generated by the Earth's spin
is greater than the planet's
gravitational pull.
These forces balance out at roughly
36,000 kilometers above the surface,
so the counterweight should be
beyond this height.
Objects at this specific distance
are in geostationary orbit,
meaning they revolve around Earth
at the same rate the planet spins,
thus appearing motionless in the sky.
The counterweight itself
could be anything,
even a captured asteroid.
From here, the tether could be released
down through the atmosphere
and connected to a base station
on the planet's surface.
To maximize centrifugal acceleration,
this anchor point should be close
to the Equator.
And by making the loading station
a mobile ocean base,
the entire system could be moved at will,
allowing it to maneuver
around extreme weather,
and dodge debris and satellites in space.
Once established, cargo could be loaded
onto devices called climbers,
which would pull packages
along the cable and into orbit.
These mechanisms would require
huge amounts of electricity,
which could be provided by solar panels
or potentially even nuclear systems.
Current designs estimate that
it would take about 8 days
to elevate an object
into geostationary orbit.
And with proper radiation shielding,
humans could theoretically
take the ride too.
So, what's stopping us from building
this massive structure?
For one thing, a construction accident
could be catastrophic.
But the main problem lies
in the cable itself.
In addition to supporting
a massive amount of weight,
the cable's material would have
to be strong enough
to withstand the counterweight's pull.
And because this tension and the force
of gravity would vary at different points,
its strength and thickness would
need to vary as well.
Engineered materials like carbon
nanotubes and diamond nano-threads
seem like our best hope
for producing materials
strong and light enough for the job.
But so far,
we've only been able to manufacture
very small nanotube chains.
Another option would be to build
one somewhere with weaker gravity.
Space elevators based on Mars or the Moon
are already possible
with existing materials.
But the huge economic advantage of
owning an Earth-based space elevator
has inspired numerous countries
to try and crack this conundrum.
In fact, some companies
in China and Japan
are already planning
to complete construction by 2050.