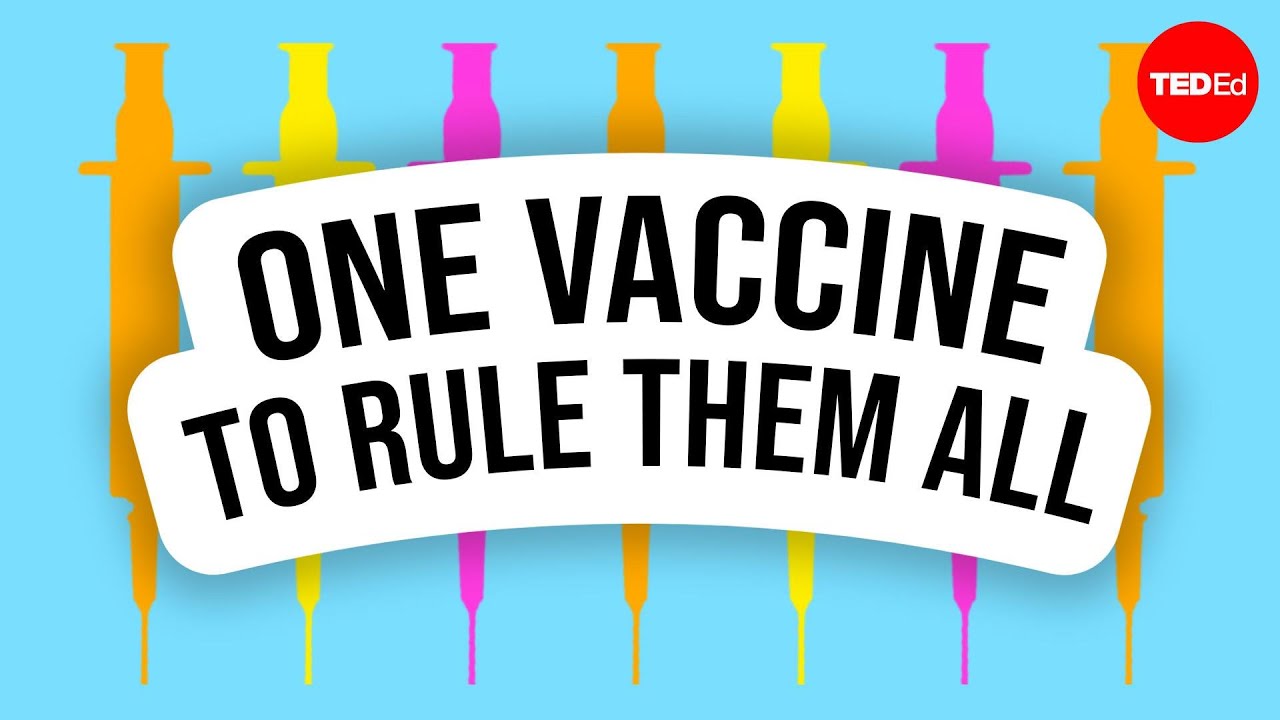
Could this technology end all viruses?
This round structure is only about
ten billionths of a meter in diameter,
but it— as well as other technologies
in the pipeline—
could be stepping stones to a monumental
public health ambition:
a single vaccine that protects you
against everything.
We’ll get back to the grand vision later,
but first,
let’s start with something that’s
being developed now:
a vaccine that would protect you
against every strain of the flu—
even ones that don’t exist yet.
Here’s one flu virus particle.
On the inside is the virus’ RNA,
and on the outside are lots and lots
of hemagglutinin proteins.
Hemagglutinin attaches to a receptor
on a human cell
and fuses the viral and human membranes,
starting the infection.
Hemagglutinin is also one of the things
your immune system recognizes
and reacts to the most.
To understand how this works,
think of hemagglutinin as a bust of 19th
century French Emperor Napoleon Bonaparte.
Croissant!
If you show Napoleon to an immune system
and say, “remember him,”
the immune system will mostly
focus on his head.
And the same is true
for the real hemagglutinin.
One way the immune system
remembers things
is by physically interacting with them.
Think of it as making plaster molds
of parts of the head:
we call these molds antibodies.
The antibodies float around your
bloodstream for a while
and then can diminish,
but blueprints on how to make them
are stored in specialized memory cells,
waiting for future Napoleons to invade.
Here’s the thing, though.
Hemagglutinin is constantly mutating.
Most mutations are subtle,
produced by single letter changes
in the virus’ RNA: like this or this.
Over time, Napoleon-slash-hemagglutinin’s
head can change enough
that our antibodies become less good
at recognizing it.
This is called antigenic drift.
Influenza is constantly drifting;
that’s one reason you have to get
a new flu shot every year.
But sometimes bigger changes happen.
An animal, usually a pig, can
get infected with, say,
a human flu and a bird flu.
And those different viruses might
infect the same cell.
If that happens, the two different viral
genomes can recombine
in tens or even hundreds of ways.
The human flu virus could
pick up a bird flu hemagglutinin
that’s never infected humans before.
This is called antigenic shift,
and if you get infected
by this version of influenza,
none of the antibodies against Napoleon's
head are going to help you.
Antigenically shifted viruses
have the potential
to infect many people very quickly,
causing epidemics and sometimes pandemics.
A truly universal flu vaccine would be
able to protect
against current flu strains
and future drifted or shifted strains.
But how do we design a vaccine
against a strain that doesn’t exist yet?
We look to the past.
There are key parts of hemagglutinin
that haven’t changed much over time
and are probably critical
to infect human cells;
these “conserved regions” could be
promising targets for universal vaccines.
But there's a problem that's hindered
classical vaccine production.
Many conserved regions are in the neck,
and it’s tough to get the immune system
to react to the neck.
Also, because influenza-like viruses have
been around
for hundreds of millions of years,
there may not be a single region
that’s common across all species
and subtypes of influenza.
But there’s promising science
in development.
Remember this?
This is a protein called ferritin;
Its normal purpose is
to store and move iron.
But it’s also the rough size and shape
of a small virus.
And if you attach viral proteins to it,
like this,
you’d have something that looks,
to an immune system, like a virus—
but would be completely harmless
and very engineerable.
Recently, scientists engineered
a ferritin nanoparticle
to present 8 identical copies
of the neck region of an H1 flu virus.
They vaccinated mice
with the nanoparticle,
then injected them with a lethal dose
of a completely different subtype,
H5N1.
All the vaccinated mice lived;
all the unvaccinated ones died.
Going one step beyond that,
there may be conserved regions
that we could take advantage of
across different-but-related
virus species—
like SARS-CoV-2, MERS,
and a few coronaviruses
which cause some common colds.
Over the past few decades,
a different part of the immune system
has come into clearer focus.
Instead of antibodies,
this part of the immune system
uses a vast array of T cells that kill,
for example,
cells that have been infected by a virus.
Vaccines that train this part
of the immune system,
in addition to the antibody response,
could provide broader protection.
A universal flu vaccine would be a
monumental achievement in public health.
A fully universal vaccine against all
infectious disease is— for the moment—
squarely in the realm of science fiction,
partially because we have no idea
how our immune system would react
if we tried to train it against hundreds
of different diseases at the same time.
Probably not well.
But that doesn’t mean it’s impossible.
Look at where medicine is today compared
to where it was two centuries ago.
Who knows what it’ll look like
in another 50 or 100 years—
maybe some future groundbreaking
technology
will bring truly universal vaccines
within our grasp.